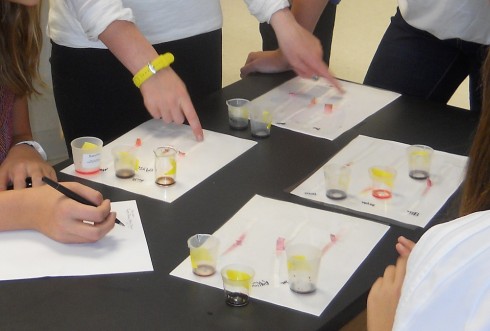
At the suggestion of Mr. Elder, I put together a Crime Scene Investigation (CSI) simulation for one of our afternoon interim activities. Sixteen students were challenged to solve a murder/mystery using simulated blood tests, fingerprinting, hair analyses, and chemical tests for drugs. And the assailants and the victims were members of the group.
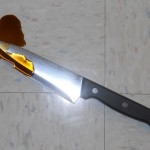
I set up the crime scene with four different lines of evidence — fingerprints, hair, blood, and drugs — and forensic methods, so I could break my students up into four groups. The students were all told that they were competing to solve the mystery; to find out what happened and who did what to whom. Without any coaxing, the groups each claimed proprietary rights one type of evidence and set about trying to solve the mystery on their own. Since none of the lines of evidence could explain everything from the crime scene they ended up having to combine what they all found.
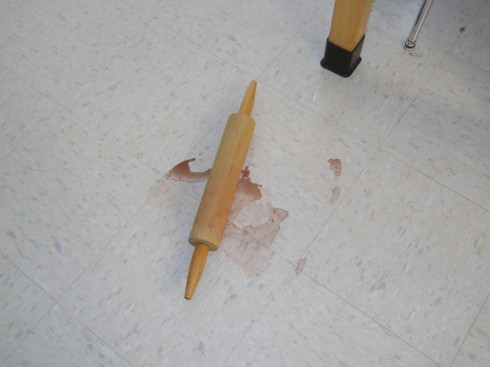
The Crime Scene
There were two weapons lying on the floor: a bloody knife and a bloody rolling pin with a hair stuck to it. On the table above the weapons were a few lines of white powder. There seemed to have been originally four lines, but one and one half of them had been used. There were fingerprints and a strand of hair next to the powder lines.
Also on the table, close to the powder, were a deck of cards (with fingerprints), a set of poker chips, a scale, and another stray hair.
Fortunately for our detectives, the fingerprints and hair had already been pulled and tagged.
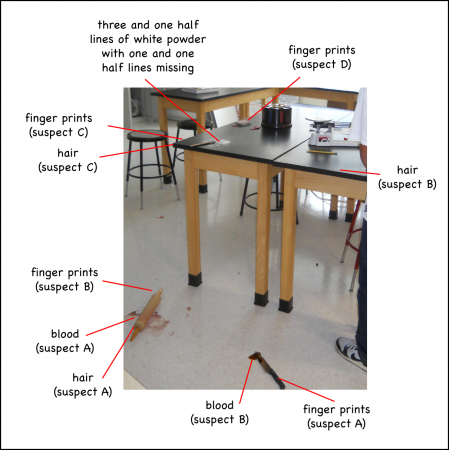
Acquiring the Evidence
It took quite a bit of effort to acquire and plant the evidence. Some of it, like the blood, was simulated, but I had to get the hair and fingerprints from the students themselves. Since the individuals who chose this activity were a self-selected fraction of the middle and high-schoolers, I wandered around the building at lunchtime at the breaks between classes trying to find one or two students who were by themselves or were in a group with others who had not chosen the CSI activity.
The crime scene setup really only requires evidence of two people, but to keep it a little more mysterious I used a little misdirection. I got five students to contribute fingerprints and hair, but told them all that they’d be the murderer. I also got one person who was not in the class to contribute as well so we’d have a set of completely mysterious evidence.
Fingerprints
I pulled fingerprints by having students rub their fingers on a black spot I’d created using a basic number 2 pencil. The student would get the black graphite on their fingers and then touch their fingertips to the sticky part of some clear tape. The fingerprints turned out quite clearly that way.
Since I did not have time to figure out how to transfer the fingerprints to the surfaces I wanted them on, I just stuck the pieces of clear tape where I wanted them in the crime scene, which also saved the detectives a bit of time and effort.
Once I told them how to get the fingerprints from their peers, the students did not need any other guidance about how to analyze the fingerprints. They took the imprinted sticky tape and stuck them to a sheet of white paper, where the black prints showed up quite nicely. Then they fingerprinted everyone in the classroom and compared, looking for whirls and swirls primarily, but also basing their conclusions on the size of the prints which they took to be indicative of gender.
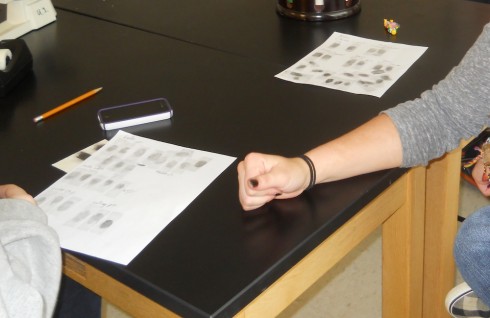
Of the four sets of prints, they were able to accurately identify the two people who were holding the knife and the rolling pin. The misidentified the one set that was from a person not in the class, and could not find the match for the last set.
Interestingly, of the four students in the group, two did most of the work while the other two wondered off to join other groups.
Hair
Hair was easy enough to collect since the students were quite happy to donate one or two for the cause. One hair per student would have been sufficient, but I kept loosing them until I just decided I’d stick them onto a piece of clear sticky tape and leave the sticky tape with hair attached at the scene of the crime.
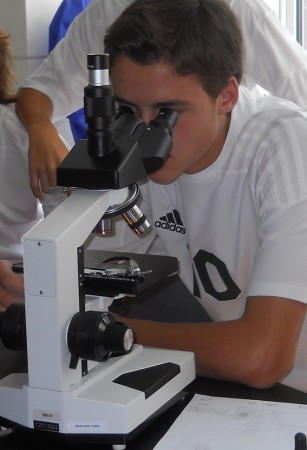
With only a little nudging, the group working on the hair realized that they could get out one of the compound microscopes to examine their specimens, and compare them to the students in the class.
One major indicator that helped with the hair identification was the length. Two of the hair samples were from girls with long hair, while one was from a fairly short haired boy. I did consider just leaving pieces of the hair as evidence, instead of whole strands, but it’s a good thing I did not since, for one reason or another, the hair group had a difficult time identifying the owners of their samples (lack of effort might have been one part of it). It did help a bit that the two major perpetrators of the crime were members of that group.
Drugs
My idea here was to simulate a drug (cocaine) deal gone bad because of a contaminated/cut product. I laid out three lines of corn starch to simulate the cocaine and one line powdered glucose in between the last two cocaine lines to represent the adulterated drug. I removed the last cocaine line and half of the glucose line to make it look like someone had been ingesting the lines and stopped part-way through.
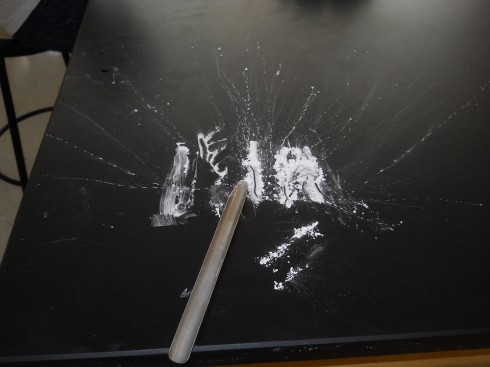
Since we’ve been testing for simple and complex carbohydrates in biology and chemistry classes I told the group testing the drugs that the test for cocaine was the same as the iodine test for starch: if you add a drop of potassium iodine to a starch solution then it turns black.
If the students had examined the drugs on the table closely enough they should have been able to see that the glucose line was different from the others; it was not as powdered (so the crystals were small but visible), and it did not clump as much as the corn starch. However, they did not, and I had to hint that they should perhaps test all the lines of powder instead of just the first sample they took.
When they discovered that one of the powder lines did not react with the potassium iodine, I told them that a common adulterant was sugar so they should perhaps test for that. One of the students remembered the Benedicts solution test, which they were able to easily conduct since I’d already had the hot water bath set up for them.
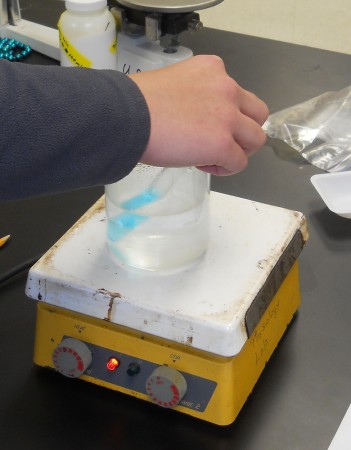
Looking through the United Nations Office on Drugs and Crime’s Recommended Methods for the Identification and Analysis of Cocaine in Seized Materials, it seems that a common test for cocaine (the Scott test) turns a solution blue when the drug is present, so the next time I try this I may have to find some tests that produce a similar color change.
Blood/DNA testing
Simulating the blood testing was one of the trickier parts of the procedure for my part since I had to keep things very organized when students started being sent to me to be blood tested.
The blood was actually a few drops of food coloring diluted into 10 ml of water. I used three drops of red in each case to try to at least get it to a somewhat blood-like color, but then in mixed in one or two other colors to get five unique blood types.
The number of drops of food coloring mixed with 10 ml of water to get the 5 blood types.
- Type 1: 3 red + 1 blue
- Type 2: 3 red + 1 green
- Type 3: 3 red + 1 yellow
- Type 4: 3 red + 1 green + 1 yellow
- Type 5: 3 red + 1 blue + 1 yellow
To match everything up with the crime scene, I assigned Suspect A to have Blood Type 2, and Suspect B to have Blood Type 4. So a sample of Blood Type 4 went on the knife, and a sample of Type 2 went on the rolling pin.
As a result, when the blood type testing group wanted to blood test everyone in the classroom, I had them send the students to me one at a time and I handed each student a small cup with a random sample of one of the Blood Types, except for the two students whose blood were on in the crime scene. With 16 students, we ended up with three or four students with each blood type.
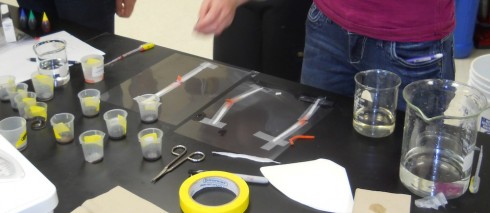
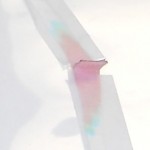
The students took their blood samples back to the testers who I’d shown a simple chromatography method. They’d cut out thin (< 1cm wide) strips of coffee filter, put a drop of the blood sample on the middle of the strip, and then taped it down to a sheet of clear overhead transparency film. Although any clear glass or plastic would have worked, the transparency film was nice because you could tape five coffee filter strips to one sheet and then loosely roll the sheet up and put one end into a partially filled beaker of water (see Figure above). Capillary action sucked the water up the strips and smeared out the blood samples so you could see its constituent colors. The method worked pretty well, and the students were able to compare the blood at the crime scene to their test results to identify the small group of people who shared the suspect blood types. It was a lot of work, and it would have taken much longer if the group doing it were not amazingly organized and worked extremely well together.
This method is more akin to blood type testing than DNA testing, which I’d have liked to simulate better, however I did not have the time to work on my chromatography method.
In Conclusion
It took a little coaxing to get them to the right conclusion in the end, but I and the student had a lot of fun solving the mystery.