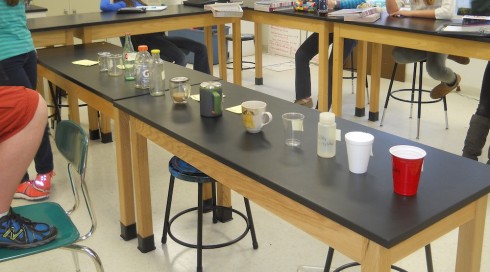
I gave the middle-schoolers twelve containers — cups, bottles, mugs, etc. — that I found around the classroom and asked them to figure out which one would keep in heat the best. In fact, I actually asked them to rank the containers because we’d just talked and read about thermal energy. This project is intended to have them learn about thermal energy and heat transfer, while discovering the advantages of the scientific method through practice.
Day 1: Observation and Deduction: When I asked them to rank that containers based on what they knew, I’d hoped that they’d discuss the thermal properties of the cups and bottles. And they did this to a certain degree, however, part of their reasoning for the numbers one and two containers, were that these were the ones I used. Indeed, since I use the double walled glass mug with the lid (container number 7) almost every day, while I only use the steel thermos-mug (container number 6) on field trips (see here for example), they reasoned that the glass mug must have better thermal properties.
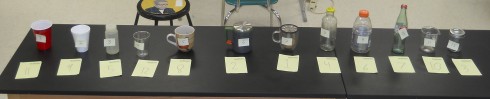
Day 2: Exploratory Science and Project Organization: On day 2, I asked the class to see how good their ranking of the containers was by actually testing them. Ever since the complex machines project where they had to choose their own objective, they’ve been wanting more independence, so I told them to pretend I was not in the room. I was not going to say or do anything to help, except provide them with a hot plate and a boiling kettle, and keep an eye out for safety.
They got to work quickly. Or at least some of them did while the other half of the class wondered around the room having their own, no-doubt important, conversations. I pulled them all back in after about half and hour to talk about what had happened. But before we discussed anything, I had them write down — pop quiz style — what their procedure was and how it could be improved. The vagueness of some of the answers made it obvious to both to me and the ones who had not been paying attention who’d actually been working on the project.
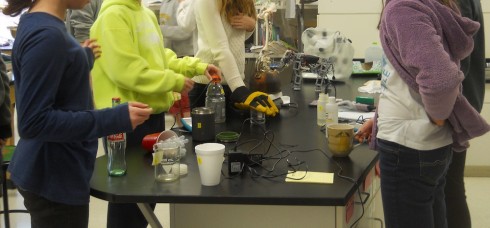
Of the ones who’d been working in the project, I brought to their attention that they’d not really spent any time planning and trying out a procedure, but they’d just jumped right in, with everyone following the instructions of the one student who they usually look to for leadership. Their procedure, while sound in theory would have benefited from a few small changes — which they did recognize themselves — and the involvement of more of the class. In particular, they were trying to check the temperature of the water every 10 seconds, but it would take a few seconds to unscrew lids, and about 5 additional seconds for the thermometer to equilibrate. They also were restricted because they were all sharing one stopwatch while trying to use multiple thermometers.
Day 3: First Iteration: Now that they’ve had a bit of trial by fire, tomorrow they’ll try their testing again. I’m optimistic that they’ve learned a lot from the second day’s experience, but we’ll see how it turns out.