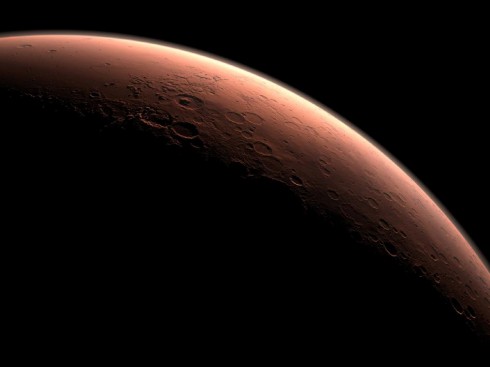
Jason Shankel has an article on how we could go about changing the surface of Mars into something humans can live on. He does an excellent job of condensing the not insignificant literature on terraforming the red planet.
Starting with an explanation of Mars’ geologic history, Shankel addresses Martyn Foggs’ list of critical challenges:
- The surface temperature must be raised
- The atmospheric pressure must be increased
- The chemical composition of the atmosphere must be changed
- The surface must be made wet
- The surface flux of UV radiation must be reduced
— Shankel (2011): How We Will Terraform Mars on io9.com.
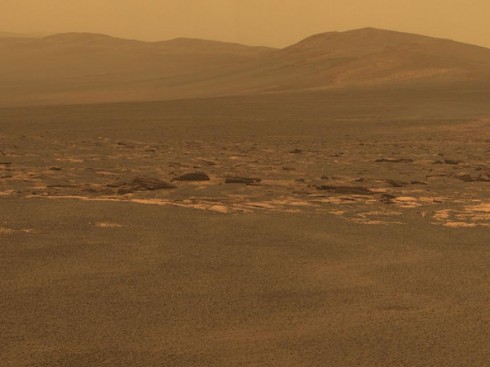
The article is expansive in its detail, provides a wonderful primer on the red planet, and demonstrates an excellent application of planetary system science (as opposed to Earth system science) to what would be an enormous geoengineering project. For example, to warm up the planet, Shankel starts with several approaches:
so how do we warm up the Martian poles? Several approaches have been suggested, from spreading dark material on the poles to lower their albedo, to industrial ice farming to good old fashioned thermonuclear detonations.
— Shankel (2011): How We Will Terraform Mars on io9.com.
He then goes into detail. Lots of detail, in a quite readable form.
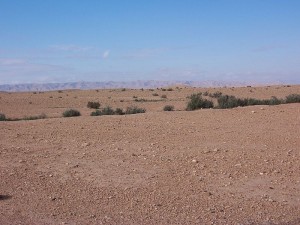